Classifying the tumor microenvironment to stratify nasopharyngeal carcinoma patients
Implication of TME in nasopharyngeal carcinoma (NPC) prognosis
In current practice, TNM classification of malignant tumors seems to be the well-recognized standard of tumor staging. It is based on the tumor burden (T), regional lymph node involvement (N), and distant metastasis (M). However, the prognostic information offered by the TNM classification is limited and cannot predict the response to treatment. Recent studies indicate that the development of NPC is affected by the tumor microenvironment (TME) and the host immune status, which is the basis for the use of TME and immunological biomarkers to predict treatment response and patient outcomes. Increasing data indicates that TME classification, especially immunological classification, has a predictive value for prognosis and could be as a useful supplement to TNM classification.
The composition of TME
Tumors are not simple aggregations of tumor cells that exist in isolation, and they also contain a complex system consisting of stromal cells, neural and neuroendocrine cells, endothelial cells (EC), immune cells, and extracellular matrix (ECMs). All these components constitute the TME, which is characterized by complex connections and interactions between cells and ECM components. These cells play important roles in tumor development, invasion, and metastasis. In this complex network, there is a large amount of information and material exchange. It is now known that chemokines, enzymes, growth factors (GF), cytokines, metabolic intermediates and metabolites of matrix proteins are present in the TME. The recruitment and activation of immune cells induced by the interaction between tumor cells and the microenvironment affect the development of tumors and the prognosis of patients. In recent years, increasing numbers of studies have focused on the TME, including large-scale analysis of stromal and immune cell types, which indicate that the TME has an important predictive value for evaluating the prognosis and response to treatment (Table 1). In addition, classification of the TME and immune cell infiltrates may be useful supplements to tumor staging and other prognostic criteria (56,57).
Table 1
Cell type | Cell marker & level | Overall outcome | Clinical outcome | References |
---|---|---|---|---|
TAMs | High CD163 expression | Undefined | No significant differences detected in pooled study | (1-6) |
High α-SMA+ CAFs | Unfavorable | Reduced OS, PFS and DMFS | (1,7) | |
CD68+ | Undefined | No significant differences detected in pooled study | (1,2,5,6,8,9) | |
High density of CD206+ M2-like TAMs | Unfavorable | Reduced OS and DFS | (6) | |
High HO-1 expression | Unfavorable | Reduced OS and PFS; poor response to radiotherapy | (3) | |
CD68+ CCL18+ | Unfavorable | Reduced OS and DFS | (6,9) | |
TILs | High CD3 TIL infiltration | Favorable | Better OS but not DFS in pooled study, despite different results in different studies | (2,10-14) |
High CD8+ TIL density | Favorable | Better OS but not PFS or DFS in pooled study, despite different results in different studies | (2,10,13-22) | |
Higher CD4/CD8 ratios | Favorable | Better 5-year DMFS, lower distant metastasis rate | (23) | |
High PD-L1 expression | Favorable | Better OS in pooled study, despite different results in different studies | (11,13,20,21,24-36) | |
AFAP1-AS1 and PD-1 co-expression | Unfavorable | Reduced OS; more likely to have distant metastasis | (37) | |
High PD-1 expression | Undefined | No statistically significant differences existed between the expression level of PD-1 in TILs and the OS in pooled study | (13,15,28,33,35,37-40) | |
CD80 and CD86 expression | Undefined | Appears to be a marker of better survival, no significance after adjustment | (41) | |
High density of NK cells | Favorable | Better OS and PFS | (15) | |
Unfavorable | Reduced 2-year OS (a higher proportion of infiltrated NK cells in the NK-high cohort co-expressed PD-1) | (38) | ||
High FoxP3/CD8+ ratios | Favorable | Better PFS in early-stage patients | (18) | |
High expression of FoxP3 and GrB | Favorable | Better OS and PFS | (18) | |
High ICOS expression | Favorable | Better OS | (42) | |
High FoxP3+ Tregs | Undefined | No significant differences detected in pooled study | (2,15,18,39,43) | |
B cells | High CD19+ B cell | Favorable | Better OS and PFS | (44) |
High memory B cells | Favorable | Better OS | (45) | |
Neutrophils | High ANC of pre-RT (>7×109/L) and a high increase (>5×109/L) of ANC during RT number of neutrophils | Unfavorable | Reduced OS | (46) |
Unfavorable | Reduced OS and PFS | (15) | ||
Mast cells | High mast cells infiltration | Unfavorable | Reduced OS and PFS | (15,47) |
Dendritic cells | High density | Favorable | Improved survival | (48-50) |
Combined | CD163−/FoxP3− | Unfavorable | Reduced OS | (43) |
CD206−/FoxP3− | Unfavorable | Reduced OS | (43) | |
CD68−/FoxP3− | Unfavorable | Reduced OS | (43) | |
NK cells combined with mast cells infiltration | Unfavorable | more recurrence or metastasis | (15) | |
Neutrophil-lymphocyte ratio | Unfavorable | Reduced OS, PFS and DMFS | (51-53) | |
Others | NO | Favorable | Better RFS | (54) |
IL-35 | Unfavorable | Reduced OS | (55) |
NPC, nasopharyngeal carcinoma; CAFs, cancer-associated fibroblasts; TAMs, tumor-associated macrophages; HO-1, high heme oxygenase-1; TILs, tumor infiltrating lymphocytes; NO, nitric oxide; NK, natural killer; RFS, recurrence-free survival; OS, overall survival; PFS, progression-free survival; DMFS, distant metastasis-free survival; DFS, disease-free survival.
Many studies (1,15,38,58,59) have suggested the crucial role of TME in the pathogenesis of NPC (NPC). EBV-positive NPC tumors are often infiltrated by a large number of leukocytes, which are involved in the early stages of cancer development (60). EBV-infected epithelial cells secrete cytokines and chemokines, or release tumor exosomes, thereby participating in the formation of the TME and the exchange of information between cells, to suppress immune surveillance and promote tumor development and metastasis (58).
ECM
The components of the TME are similar to normal or inflamed tissue, including the ECM, non-immune/inflammatory stromal cells, and immune cells. The ECM is composed of hyaluronic acid, proteoglycans and other matrix proteins, along with cytokines, hormones and GF secreted by cells nearby (61). The ECM can influence tumor development by altering the microenvironment (57). For example, the ECM can provide a hypoxic or acidic microenvironment, which gives a survival advantage to tumor cells that obtain energy through anaerobic glycolysis compared to normal cells that require aerobic oxidation. Additionally, the abundant ECM in the TME can promote tumor growth by activating the pro-survival phosphoinositide 3-kinase (PI3K) signaling pathway (56). Moreover, interactions between the ECM and lymphocytes have a key impact on the movement and positioning of immune cells (62), which can help tumor cells evade immune surveillance. For example, ECM deposition and increased density can reduce lymphocyte replacement, limit T cell movement, and thereby suppress the anti-tumor effect of the body’s immune response (56). A recent study shows that, in the NPC microenvironment, ECM is one of the key components mediating immunosuppressive functions. The main ECM components, such as collagen and fibronectin, are beneficial to angiogenesis and inhibit tumor cell apoptosis. In addition, the tumor parenchymal barrier constituted by the ECM is a physical protective barrier for tumor cells, which can attenuate T cell infiltration and drug penetration (63).
Stromal cells
Stromal cells mainly include vascular ECs, pericytes, mesenchymal stem cells (MSCs), fibroblasts, neural/neuroendocrine cells, and adipocytes. Perivascular cells, EC, and smooth muscle cells are the main components of blood vessels. A study of breast cancer tumors (64) found that neovascular tips are enriched with active TGF-β1 and periostin, which can promote growth. ECs are involved in tumor proliferation, invasion, and metastasis, while pericytes can prevent lymphocyte infiltration and effectively down-regulate the inflammatory response at the tumor site (65). MSCs can differentiate into a variety of other stromal cells, such as fibroblasts, pericytes, adipocytes, osteocytes, and chondrocytes. They also have immunoregulatory functions, such as secreting cytokines, GF and immune receptors, regulating the tissue microenvironment (66). For example, In the NPC microenvironment, fibroblasts can secrete a variety of GF that promote tumor progression or immunosuppression, including EGF, FGF, TGF-β, CSF, and IGF1 (67).
Cancer-associated fibroblasts (CAFs)
As the main non-inflammatory stromal cell type in the TME, CAFs are heterogeneous cells from multiple sources that also synthesize the ECM. Studies suggest that CAFs may also hinder the anti-tumor immune response (68,69). By producing TGF-β, malignant cells can activate adjacent CAFs, which in turn release large amounts of interleukins and chemokines, such as IL-1, IL-6, IL-8, IL-22, CXCL and CCL (70). In addition, CAFs can enhance tumor progression by promoting angiogenesis or through the interaction of different cytokines and chemokines with inflammatory and neuroendocrine cells. The accumulation of CAFs is usually associated with a poor prognosis (56). A study of 154 NPC patients found a significant relation between the M2 tumor-associated macrophages (TAMs) and CAFs density, which can be used as an independent predictor of patient outcomes. This study stratified the patients into three groups based on the CD163 and α-SMA expression levels, which was further confirmed by a validation test (1).
Immune and inflammatory cells
A large number of immune and inflammatory cells are also present in the TME, such as natural killer (NK) cells, T cells, B cells, and dendritic cells (DCs). In addition, other myeloid precursor cells such as neutrophils, macrophages and mast cells infiltrate the TME attracted by the inflammatory chemokines produced by tumor cells. The invasive margin of cancer may include tertiary lymphatic structures (TLS), which have similar characteristics to peripheral lymph node tissue. TLS act as a functional area to induce immune cell activation and differentiation, where plasma cells can produce a large number of specific antibodies, which is related to the induction of the adaptive immune response (71). From the perspective of tissue distribution, immune cells have a specific enrichment patter in the tumor, which is also closely related to their functions. For example, immature DCs are mainly located in the central area of the tumor, while mature DCs penetrate into the edge that is close to T cells. B cells exist in the TLS and infiltration margins, while NK cells are scattered in the stroma and infiltration margins (characteristics of tertiary lymphoid structures in primary cancers). In addition, in the TME of NPC, B lymphocytes, NK cells, monocytes, and neutrophils can also be found (72).
NK and NKT cells
As part of the innate immune system, NK cells (CD56+/CD3−) play an important role in preventing infection and cancer. NKT (CD56+/CD3+) cells, like its name, share multiple markers of both NK cells and T lymphocytes, and express NK group 2D (NKG2D) receptors (73). In terms of cancer prognosis, NK cell infiltration showed inconsistent results. A study of 9 types of immune cells in 197 NPC patients showed that a high density of NK cells is correlated with favorable OS and PSF (15). However, another study suggested that patients with higher levels of tumor-infiltrating NK cells (PD-1+/NKhigh) have a poorer 2-year survival (38). This controversy may partly be due to the presence of different subtypes of NK cells in NPC. One case report described an advanced NPC patient with intracranial metastases that progressed during conventional therapy. After NK cell transfer therapy combined with chemoradiotherapy, the patient maintained long-term tumor control (74).
Tumor infiltrating lymphocytes (TILs)
TILs are a group of T cells that surround malignant cells and consist mainly of CD4+ and CD8+ T cells (75). CD4+ T cells include CD4+ Th cells and CD4+ CD25+ FoxP3+ Treg cells, which mediate anti-tumor immune response and suppress anti-tumor immunity, respectively (76,77). There are many infiltrating lymphocytes in NPC tumor stroma including anti-LMP1 and -LMP2 cytotoxic T cell precursors. However, these precursors do not kill tumor cells normally, which may due to the immunosuppressive microenvironment (72). Another study shows that among infiltrating lymphocytes, CD3+ T lymphocytes usually account for more than 50% (59). The massive infiltration of leukocytes has been shown to be a shared characteristics of primary NPC tissues, but it is less frequently presented in metastatic lesions (78). This phenomenon suggests that the infiltration of leukocytes may have a more important role in the NPC development, whereas lymphocyte infiltration may have less effects on late and metastatic lesions. It is worth noting that in NPC, lymphocyte infiltration does not seem participate in eliminating tumor cells, but instead promotes tumor development (72). There is abnormal T cell activity in patients with undifferentiated NPC: peripheral CD4+ T cells of these patients are deficient in producing IL-2, whereas higher level of IL-10 were detected, which suggests a Th-1/Th-2 imbalance (79). TILs expanded under IL-2low conditions lack cytotoxic activity, so they can neither lyse autologous EBV-infected NPC cells nor produce IFN-γ (80). Although EBV-specific cytotoxic T lymphocytes (CTLs) from the patient's blood can be reactivated, these activated CTLs will be selectively non-functional at the NPC tumor site (80). Thus, to reverse the immunosuppressive effect of the TME may provide an option for effective NPC treatment.
Studies have shown that in Hodgkin lymphoma, the expression of EBNA1 induces the production of CCL20 and the migration of Treg cells (81). High levels of CCL20 (MIP-3α) have also been detected in NPC patients. This may be the cause of Treg migration to the TME (82,83). The PBMC and TIL of NPC patients were found to contain a higher proportion of CD25+ Treg cells than healthy controls (83). In addition, interferon (IFN)-γ, IL-10, IL-8 and IL-6 levels were elevated, whereas IL-2 levels were decreased in NPC biopsies, which may contribute to immune cell recruiting and regulation of cellular immunity (80,82,84,85).
In 2018, a cohort study of 1,490 NPC samples investigated whether TIL density can be used to assess the prognosis of patients with NPC. This study proved that high TIL infiltration is significantly associated with longer overall survival (OS), disease-free survival (DFS), distant metastasis-free survival (DMFS), and local recurrence-free survival, and it can be used as an independent prognostic indicator for patients with non-disseminated NPC (86). In addition, a study conducted in 2016 involving 719 patients suggested that the CD4/CD8 ratio can effectively predict the distant metastasis risk, whereby higher CD4/CD8 ratio correlated with better 5-year DMFS. High CD3+ or CD8+ T cell infiltration predicted better OS, but not DFS in this study (2).
B cells
B cells have also been found in the TME. B cells can aggregate at tumor margins or into complex immune aggregates, which are often related to the formation of ectopic lymphoid tissues, and have a positive effect on the differentiation of tumor-specific B- and T-cell responses (87,88). However, the prognostic value of B cell infiltration in NPC is not well-established. CXCL13, which interacts with the CXCR5 receptor in B cells, is considered to be one of the most effective chemoattractants for B cells. As a consequence, the expression of CXCL13 may affect tumor progression by recruiting more B cells to the tumor site (89,90). The increase of CXCL13 expression at the tumor site has been shown to be associated with better survival in other tumors, such as colorectal and breast cancer (91,92). In NPC, a recent study on PD-1+CXCR5−CD4+Th-CXCL13 cell subsets suggested that an increase in Th-CXCL13 cell subsets is associated with better prognosis in NPC patients, while the infiltration of CD38+ plasmablasts and CD138+ plasma cells in the TME is associated with better survival outcomes, suggesting a cooperative role of B cells in the TME (93).
DC cells
DC cells are considered to act as a bridge between innate and adaptive immunity. They are also the pioneer cells that migrate the fastest to the tumor site due to attraction by chemokines (CXCL12 and CXCL8), vascular endothelial growth factor (VEGF) and hepatocyte growth factor (HGF), and antimicrobial peptides (β-defensin) that are secreted by tumor and stromal cells. After immature DCs interact with an antigen, they will migrate to the secondary lymphoid organs where lymphocytes are enriched, and act as antigen presenting cells (APCs) to further induce specific immune responses (94,95). Although DCs may be present in NPC, there is not much research on their role in this malignancy. A study of tumor samples from untreated NPC patients demonstrated that CD207 expression was higher in CD1c+ DCs from NPC lesions, comparing to CD141+ and CD141−CD1c− DCs, and CD123+ DCs, suggesting that CD207+ DCs might serve as therapeutic targets for cross-presentation of tumor antigens (96). Studies have shown that high infiltration of DCs, believed to mediate T-cell interactions, is association with improved patient outcomes (48-50).
TAMs
The EBV-related genes and cytokines expressed by NPC cells also play an important role in promoting the secretion of pro-inflammatory cytokines. LMP1 encoded by EBV can induce CXCL-10 expression through NF-κB signaling (97). IL-18 can be released by NPC cells, forming a positive feedback loop to exacerbate leukocyte infiltration and inflammation (97,98). Under the circumstances, CD68+ macrophages can be stimulated to produce IL-12 and IL-18, and further stimulate both T and NK cells. Once activated, macrophages can differentiate into M1 and M2 phenotypes (99). M1 macrophages have strong antigen presentation ability, and they participate in antitumor immune response along with Th1 cells. By contrast, the immune-suppressive M2 macrophages produce IL-10, which is essential for initiating Th2-type responses and suppressing CTL responses. In the process of tumor development, the pre-invasive TME has anti-tumor properties, mainly including M1 and Th1 cells, leading to the production of inducible NO synthase (iNOS), IFNγ, and IL-12. The transition toward an aggressive TME is marked by the emergence of tumor-promoting properties, characterized by the transition from Th1 to Th2 cells and M1 to M2 cells (100). In EBV-positive NPC biopsies, CD68+ TAM and FoxP3+ Treg cells was found to be increased in EBV-positive NPC specimens. Treg cells are likely to promote primary NPC cells migration by RANKL, which leads to metastasis and poor survival (43). However, in a systematic analysis conducted in 2021 on the prognostic value of macrophage and lymphocyte infiltration, there was no prognostic significance of CD68+ or CD163+ macrophages (2). However, a large cohort study is needed to confirm these findings. A recent study of primary antioxidant enzyme heme oxygenase-1 (HO-1), showed that it plays a crucial role in the polarization of M2 macrophages, suggesting that its expression is highly correlated with CD163+ status. Moreover, HO-1 exhibited higher accuracy in the prognosis of NPC patients than CD163 (3).
Neutrophils
Neutrophils are one of the first immune cells to be recruited to inflamed tumor tissues (101). Neutrophils can form a network structure and release reactive oxygen species (ROS) that can damage DNA. Neutrophil extracellular traps (NETs) can be formed in the TME, where they play a tumor-promoting role (102,103). The EBV lytic trans-activator Zta was found to upregulate prostaglandin E2 (PGE2) and granulocyte-macrophage colony stimulating factor (GM-CSF) (104) to promote the production of IL-10 from neighboring monocytes in the TME (104), effectively inhibiting the cytotoxic function of activated CD8+ T cells (105). In addition, Zta can induce NPC cells to express IL-8, which is a powerful chemical attractant for neutrophils (106).
A survey of 1,753 patients with NPC showed that a high absolute neutrophil count (ANC) before radiotherapy (>7×109/L) and large ANC increase during radiotherapy (>5×109/L) were significantly correlated with poor OS and can be regarded as an independent prognostic factor (46). Similarly, high infiltration of neutrophils was found to be associated with poor prognosis (15). Another study found the neutrophil-to-lymphocyte ratio (NLR) was correlated with T stage in stage II NPC patients, whereby high NLR was significantly associated with poor prognosis. The study suggests that NLR can be used as an independent prognostic indicator in stage II NPC patients (107).
Other factors
In addition to the above-mentioned cellular components, the presence of cytokines and other substances in tumor tissues also has a potential prognostic value. High expression of IL-35, which is correlated with EBV-induced gene 3 (EBI3) and IL-12p35, was found to be associated with the progression of NPC, and patients with high EBI3 or p35 staining were more likely to have a more advanced tumor and shorter survival. By contrast, nitric oxide (NO) was found to act as a key mediator of tumor growth, and it is involved in the IL-6/NOS2 inflammatory signal pathway. It was also found that elevated levels of serum nitrite are linked to better recurrence-free survival (54).
Application of scRNA-seq in the evaluation of survival and prognosis in NPC
High PD-L1 expression of tumor cells and abundant infiltration of non-malignant lymphocytes (about 50% of samples with >70% stromal TILs or >10% intratumoral TILs) are major characteristics of NPC (11,13,21,29,86). Several important single-arm trials evaluating anti-PD-1 monoclonal antibodies in recurrent or metastatic NPC showed promising clinical outcomes (108-110). However, PD-1 inhibition benefits only a subset of patients, which proposes challenge of identifying biomarkers associated with immunotherapy response for different patient subgroups. Unfortunately, no strong evidence currently supports the correlation between response rate to anti-PD-1 monoclonal antibodies and known biomarkers such as expression levels of typical marker genes in tumor or immune cells. Nonetheless, more large-scale clinical studies and preclinical investigations are warranted to identify and validate potential therapeutic biomarkers and major subtypes in TME (e.g., TIL infiltration level, immune gene signatures, immune molecular subgroups) (111-114), which might provide an insight into NPC treatment response or resistance.
Understanding intratumoral heterogeneity is one of the greatest difficulties in cancer biology, diagnosis, and therapy (115). Developments in scRNA-seq technologies have now provided a powerful tool to explore different cell subpopulations in the TME, dissect the pathogenic hierarchies, identify novel driver genes, and illustrate the infiltrating-immune surveillance system that determines tumor progression (116-119) (Figure 1). The comprehensive single-cell transcriptome atlas of the multicellular ecosystem of the NPC TME (TME) lays a new foundation for the development of diagnosis and precision therapies (120).
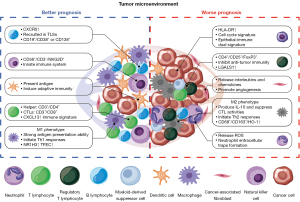
Subtype-specific gene signatures
By revealing the cellular heterogeneity of the TME by scRNA-seq, several intratumoral and immune subtype-specific gene signatures have been identified as potential biomarkers for the prognosis of NPC. Recently, the role of cell cycle regulation in tumorigeneses and progression has been well characterized in NPC that the cell cycle status of malignant cells could be an important factor contributing to the heterogeneity of tumors (121-125), and several reagents targeting various cell-cycle regulators have been tested in preclinical trials and clinical therapy in cancer patients (126-128). Zhao et al. observed that some cell cycle genes related to cell proliferation were significantly increased in EBV-positive NPC cells (129). Furthermore, survival analyses from another study revealed that high cell cycling scores significantly predicted poor survival in a clinical NPC patient cohort, which suggests that the cell cycling signatures may reflect a highly proliferative and aggressive status, reflecting the aggressiveness of NPC (130). Overall, cell cycle modulators are considered as attractive potential therapeutic targets in these NPC patients. Since radiotherapy, one of the most common treatments for NPC, induces cell cycle arrest and apoptosis (131), the combination of radiotherapy and chemotherapy with cell cycle inhibitors may be a more effective treatment modality for EBV-positive NPC. Intriguingly, the cell cycling signature did not predict survival in head and neck squamous cell carcinoma (HNSCC) (130), which means that cell cycling signatures may be a tumor-specific phenomenon of NPC.
Immune cells, particularly T and B lymphocytes, play important roles in the immunological surveillance that can potentially eliminate tumor cells (132-134). Previous studies have shown that higher levels of particular lymphocyte subpopulations in the peripheral blood were associated with tumor development and poor prognosis in NPC patients (133,135). It was also reported that an early clinical N stage and high CD4/CD8 ratio may serve as better predictors of outcomes in patient s with distant metastasis (23). Additionally, an immune-enriched subtype, identified through virtual microdissection of gene expression profiles, was found to predict the clinical outcomes and immunotherapy responses of suitable patients (136). However, there are also reports that CD8 and PD-L1 expression levels of tumor-infiltrating immune cells are not correlated with clinical outcomes (20,21). Consequently, more evidence is needed to identify biomarkers predicting the effectiveness of immune checkpoint inhibitors (ICIs) in NPC.
Heterogeneous immune cells have been characterized using transcriptional profiling at single-cell resolution in several cancers, revealing that certain subtypes of immune cells and gene signatures in the TME are important for tumor progression and sustained treatment responses (129). Profound infiltration of lymphocytes has been observed in histological biopsies of NPC. Moreover, a high density of TILs was associated with favorable survival outcomes in patients with NPC (129). Furthermore, Gong et al. found that CXCL13 and LGALS1 were specifically upregulated in NPC-derived T cells, illustrating the molecular variation between T cell infiltrates in the tumor and non-malignant microenvironments (137). Higher expression of CXCL13, together with a higher fraction of CXCR5+ B cells, was found to be correlated with better progression-free survival (PFS) in NPC patients, suggesting the CXCL13-high T cells might be of great importance in tumor immune modulation. While LGALS1 was reported to have an immunoregulatory function in various immune cells, a higher level of immunosuppressive Tregs, instead of resting Tregs, was identified in LGALS1-high patients, and higher expression of LGALS1 was associated with poor PFS, indicating that LGALS1 might be a key regulator in Treg activation. Among other immune cell signatures, high expression of the specific genes of macrophages, DCs, DC1, NK cells, and plasma cells significantly predicted a favorable prognosis in NPC (130).
Interestingly, the epithelial-immune dual feature of NPC tumor cells, which is mainly characterized by the expression of IFN response genes, has been defined recently (138). Higher expression of HLA-DR on tumor cells was correlated with poorer OS, indicating that the epithelial–immune dual feature was closely associated with the prognosis in NPC. The tumor cells with high immune scores (ISs) increased the expression of PD-1, LAG-3, TIM-3 in both CD4+ and CD8+ cells, while reducing IFN-γ secretion in TILs, indicating that IS-high tumor cells may induce the exhaustion of TILs. These findings suggest that the ISs of tumor cells can serve as a potential biomarker for assessing the prognosis of NPC patients. As an alternative form of molecular subtyping, patient stratification based on the TME hierarchy can serve as an indicator that is closely associated with treatment responses and prognosis in NPC.
In addition to traditional well-characterized immune cells and tumor cells, some cell types with low abundance in the TME of NPC may nevertheless be of great importance. Of note, Chen et al. found increased expression of NR1H3 and TFEC, which facilitate the differentiation and maturation of monocyte-derived macrophages (130). Further survival analyses revealed an association between high expression levels of NR1H3 and TFEC and improved outcomes in NPC patients. These results support the idea that NR1H3 and TFEC may promote antitumor immunity in NPC.
By dissecting tumor heterogeneity using scRNA-seq, functional cell types that are associated with tumor state and clinical outcomes of NPC patients can be identified. Subtype-specific gene signatures may be a strong indicator for survival and prognosis. Importantly, the relationship between NPC-specific characteristics and patient stratification and survival have been well validated, which makes great contribution to the development of personalized prognostic and therapeutic approaches. However, whether the gene signatures identified based on specific subtypes can be simply applied to bulk NPC samples still needs further validation in larger cohort studies.
Deconvolution based on the cell-type composition
Gene expression analyses of bulk tissues ignore the cell type composition as an important confounding factor, resulting in a loss of signals from rare cell types. However, scRNA-seq has limited value in clinical practice due to its high cost and long processing time. Thus, using computational deconvolution methods to infer the abundance of different cell types and/or cell type-specific expression profiles in heterogeneous samples without performing physical cell sorting is of great significance for the broader clinical application of these findings (139).
Based on the computational deconvolution methods, Gong et al. leveraged a RNA sequencing data of 88 NPC patients with clinical information for deconvolution using an annotated signature matrix containing 733 representative genes for selected major subpopulations of T cells, B cells and myeloid cells to estimate the abundance of immune-cell subtypes (137). The patients were further clustered into three groups based on immune-cell abundance according to the activation status of tumor immune microenvironment (TIME). The results indicated that a higher fraction of exhausted T cells, plasma B cells, DCs and macrophages was correlated with a better prognosis in NPC patients. On the other head, abundance of double-negative B cells and MDSCs in NPC patients was predictive of worse PFS. Moreover, other than cell type proportions, marker gene signatures such as low T-immune scores or inactivated TIME were found to be associated with worse prognosis (137).
Instead of adopting selected major subpopulations in a certain cell-type, Jin et al. partitioned 140 primary NPC tumors from treatment-naïve patients into 5 groups (G1-G5) according to their notable differences in NPC TME composition and analyzed their correlation with PFS. Tumors from G4 and G5 exhibiting relatively lower immune cell-associated signatures and were associated with a shorter PFS outcome, whereby the G5 subgroup with a higher expression of the fibroblast-associated signature had an even worse prognosis. Tumors from G1, G2 and G3 had relatively higher abundance of immune cells and presented a better prognosis. Interestingly, the G2 subgroup with a remarkably higher fraction of fibroblasts was correlated with a shorter PFS than the G1 and G3 subgroups. These data indicated that the affiliation among several major cell-types mutually influenced tumor progression (138).
Currently, there is still a lack of representative clinical models for prognostic risk stratification, survival prediction and therapeutics and drug treatment evaluation in NPC. The incorporation of functional modules into the single cell-based devolution method enhanced its reliability as an integrated and feasible model to classify cancer subtypes, as well as predict the survival and therapeutic outcomes, but it is still of great necessity to validate these findings in larger clinical cohorts.
Conclusions and outlook
Tumor formation involves the co-evolution of neoplastic cells together with ECM, stromal cells, tumor vasculature and immune cells. Tumor progression and eventual metastasis is not determined solely by tumor cells, but also by the alterations of different cell types in TME. Heterologous cell types within tumors can actively influence therapeutic response and shape resistance, therefore evaluating tumors as complete organs together with crucial cell components in TME is of vital importance.
More and more studies (130,137,138) have corroborated the idea that specific NPC-specific signatures were closely associated with patient survival, indicating that the relative abundance of various stromal subpopulations and immune activation status in NPC patients directly influence tumor development and treatment outcomes. A pharmacogenomics-based precision medicine approach by integrating genomics with a drug sensitivity test has been conducted recently to investigate the correlation between biological features of different subtypes of NPC and their responses to therapeutic drugs (140), enlightening the application prospects of genomics-based precision medicine. The emerging scRNA-seq studies of NPC provide new opportunities for identifying novel signatures as prognostic biomarkers and pathogenic cell components as therapeutic targets. The minor subpopulations residing in the NPC microenvironment might also influence the clinical outcomes (63,114). Hence, single-cell sequencing technology is an important and powerful tool for the in-depth identification and characterization of minor subpopulations within the heterogeneous tumor mass.
Since scRNA-seq became more and more conventional, technological advancements with unprecedented cellular resolution such as single cell spatial transcriptomics, advanced tissue histology approaches and new molecular immune profiling methods provides useful tools to dissect the complex cell-to-cell interplays within TME, which may support better theoretical basis during immunotherapy and drug development. Spatial transcriptomic approaches are addressing the main drawback of scRNA-seq that the significant intra-tissue information (shape, cell-cell and cell-matrix interactions) after dissociation was abandoned (141). Recent study suggested that compartmentalization of potential immune suppression and pathogenic subpopulation-enriched gene networks revealed by single cell spatial transcriptomics may be associated with tumor subclones and tumor progression in squamous cell carcinoma patients (142). We are entering a new era of precision immunotherapy that utilizes the unearthing of predictive biomarkers, understanding of cell components in microenvironment, and design of combination therapies with better clinical application prospects. Hopefully, with the development of powerful techniques, comprehensive workflows will be established to investigate the biological mechanisms underlying its pathogenesis, refine screening and staging strategies, identify biomarkers related to prognostic risk stratification, optimize treatment strategies for the different patient subgroups, and develop novel therapeutics and drug treatment.
Acknowledgments
Funding: This work was financially supported by the grant from the Natural Science Foundation of China (82072982).
Footnote
Provenance and Peer Review: This article was commissioned by the Guest Editors (Maria Li. Lung and Lawrence S. Young) for the series “NPC Biomarkers” published in Annals of Nasopharynx Cancer. The article has undergone external peer review.
Peer Review File: Available at https://anpc.amegroups.com/article/view/10.21037/anpc-21-10/prf
Conflicts of Interest: The authors have completed the ICMJE uniform disclosure form (available at https://anpc.amegroups.com/article/view/10.21037/anpc-21-10/coif). The series “NPC Biomarkers” was commissioned by the editorial office without any funding or sponsorship. MSZ serves as unpaid editorial board member of Annals of Nasopharynx Cancer from January 2021 to December 2022. The authors have no other conflicts of interest to declare.
Ethical Statement: The authors are accountable for all aspects of the work in ensuring that questions related to the accuracy or integrity of any part of the work are appropriately investigated and resolved.
Open Access Statement: This is an Open Access article distributed in accordance with the Creative Commons Attribution-NonCommercial-NoDerivs 4.0 International License (CC BY-NC-ND 4.0), which permits the non-commercial replication and distribution of the article with the strict proviso that no changes or edits are made and the original work is properly cited (including links to both the formal publication through the relevant DOI and the license). See: https://creativecommons.org/licenses/by-nc-nd/4.0/.
References
- Yu Y, Ke L, Lv X, et al. The prognostic significance of carcinoma-associated fibroblasts and tumor-associated macrophages in nasopharyngeal carcinoma. Cancer Manag Res 2018;10:1935-46. [Crossref] [PubMed]
- Liu W, Chen G, Zhang C, et al. Prognostic significance of tumor-infiltrating lymphocytes and macrophages in nasopharyngeal carcinoma: a systematic review and meta-analysis. Eur Arch Otorhinolaryngol 2022;279:25-35. [Crossref] [PubMed]
- Huang J, Wan B, Li S, et al. High expression of heme oxygenase-1 in tumor-associated macrophages characterizes a poor-prognosis subtype in nasopharyngeal carcinoma. Aging (Albany NY) 2021;13:5674-85. [Crossref] [PubMed]
- Huang H, Liu X, Zhao F, et al. M2-polarized tumour-associated macrophages in stroma correlate with poor prognosis and Epstein-Barr viral infection in nasopharyngeal carcinoma. Acta Otolaryngol 2017;137:888-94. [Crossref] [PubMed]
- Deng R, Lu J, Liu X, et al. PD-L1 Expression is Highly Associated with Tumor-Associated Macrophage Infiltration in Nasopharyngeal Carcinoma. Cancer Manag Res 2020;12:11585-96. [Crossref] [PubMed]
- Chen YL. Prognostic significance of tumor-associated macrophages in patients with nasopharyngeal carcinoma: A meta-analysis. Medicine (Baltimore) 2020;99:e21999. [Crossref] [PubMed]
- Chen J, Yang P, Xiao Y, et al. Overexpression of α-sma-positive fibroblasts (CAFs) in Nasopharyngeal Carcinoma Predicts Poor Prognosis. J Cancer 2017;8:3897-902. [Crossref] [PubMed]
- He C, Zhuang H, Zhang W. Immunocyte reaction in stage III nasopharyngeal carcinoma in relation to biological findings and prognosis. Zhonghua Bing Li Xue Za Zhi 1999;28:115-8. [PubMed]
- Huang D, Song SJ, Wu ZZ, et al. Epstein-Barr Virus-Induced VEGF and GM-CSF Drive Nasopharyngeal Carcinoma Metastasis via Recruitment and Activation of Macrophages. Cancer Res 2017;77:3591-604. [Crossref] [PubMed]
- Al-Rajhi N, Soudy H, Ahmed SA, et al. CD3+T-lymphocyte infiltration is an independent prognostic factor for advanced nasopharyngeal carcinoma. BMC Cancer 2020;20:240. [Crossref] [PubMed]
- Zhu Q, Cai MY, Chen CL, et al. Tumor cells PD-L1 expression as a favorable prognosis factor in nasopharyngeal carcinoma patients with pre-existing intratumor-infiltrating lymphocytes. Oncoimmunology 2017;6:e1312240. [Crossref] [PubMed]
- Ben-Haj-Ayed A, Moussa A, Ghedira R, et al. Prognostic value of indoleamine 2,3-dioxygenase activity and expression in nasopharyngeal carcinoma. Immunol Lett 2016;169:23-32. [Crossref] [PubMed]
- Ono T, Azuma K, Kawahara A, et al. Prognostic stratification of patients with nasopharyngeal carcinoma based on tumor immune microenvironment. Head Neck 2018;40:2007-19. [Crossref] [PubMed]
- Wang YQ, Chen L, Mao YP, et al. Prognostic value of immune score in nasopharyngeal carcinoma using digital pathology. J Immunother Cancer 2020;8:e000334. [Crossref] [PubMed]
- Lu J, Chen XM, Huang HR, et al. Detailed analysis of inflammatory cell infiltration and the prognostic impact on nasopharyngeal carcinoma. Head Neck 2018;40:1245-53. [Crossref] [PubMed]
- Chen TC, Chen CH, Wang CP, et al. The immunologic advantage of recurrent nasopharyngeal carcinoma from the viewpoint of Galectin-9/Tim-3-related changes in the tumour microenvironment. Sci Rep 2017;7:10349. [Crossref] [PubMed]
- Ooft ML, van Ipenburg JA, Braunius WW, et al. Prognostic role of tumor infiltrating lymphocytes in EBV positive and EBV negative nasopharyngeal carcinoma. Oral Oncol 2017;71:16-25. [Crossref] [PubMed]
- Zhang YL, Li J, Mo HY, et al. Different subsets of tumor infiltrating lymphocytes correlate with NPC progression in different ways. Mol Cancer 2010;9:4. [Crossref] [PubMed]
- Ogino T, Moriai S, Ishida Y, et al. Association of immunoescape mechanisms with Epstein-Barr virus infection in nasopharyngeal carcinoma. Int J Cancer 2007;120:2401-10. [Crossref] [PubMed]
- Chan OS, Kowanetz M, Ng WT, et al. Characterization of PD-L1 expression and immune cell infiltration in nasopharyngeal cancer. Oral Oncol 2017;67:52-60. [Crossref] [PubMed]
- Larbcharoensub N, Mahaprom K, Jiarpinitnun C, et al. Characterization of PD-L1 and PD-1 Expression and CD8+ Tumor-infiltrating Lymphocyte in Epstein-Barr Virus-associated Nasopharyngeal Carcinoma. Am J Clin Oncol 2018;41:1204-10. [Crossref] [PubMed]
- Minichsdorfer C, Oberndorfer F, Krall C, et al. PD-L1 Expression on Tumor Cells Is Associated With a Poor Outcome in a Cohort of Caucasian Nasopharyngeal Carcinoma Patients. Front Oncol 2019;9:1334. [Crossref] [PubMed]
- Tao CJ, Chen YY, Jiang F, et al. A prognostic model combining CD4/CD8 ratio and N stage predicts the risk of distant metastasis for patients with nasopharyngeal carcinoma treated by intensity modulated radiotherapy. Oncotarget 2016;7:46653-61. [Crossref] [PubMed]
- Huang ZL, Liu S, Wang GN, et al. The prognostic significance of PD-L1 and PD-1 expression in patients with nasopharyngeal carcinoma: a systematic review and meta-analysis. Cancer Cell Int 2019;19:141. [Crossref] [PubMed]
- Hu B, Sun M, Wang Z, et al. Prognostic Value of Programmed Cell Death-Ligand 1 Expression in Tumor-Infiltrating Lymphocytes and Viral Load in Peripheral Blood Mononuclear Cells for Epstein-Barr Virus-Positive Nasopharyngeal Carcinoma. Clin Chem 2020;66:1219-27. [Crossref] [PubMed]
- Zhang J, Fang W, Qin T, et al. Co-expression of PD-1 and PD-L1 predicts poor outcome in nasopharyngeal carcinoma. Med Oncol 2015;32:86. [Crossref] [PubMed]
- Chang AMV, Chiosea SI, Altman A, et al. Programmed Death-Ligand 1 Expression, Microsatellite Instability, Epstein-Barr Virus, and Human Papillomavirus in Nasopharyngeal Carcinomas of Patients from the Philippines. Head Neck Pathol 2017;11:203-11. [Crossref] [PubMed]
- Cao C, Wei Q, Tang X, et al. PD-1 and PD-L1 in locoregionally advanced nasopharyngeal carcinoma: Substudy of a randomized phase III trial. Head Neck 2019;41:1427-33. [Crossref] [PubMed]
- Lee VH, Lo AW, Leung CY, et al. Correlation of PD-L1 Expression of Tumor Cells with Survival Outcomes after Radical Intensity-Modulated Radiation Therapy for Non-Metastatic Nasopharyngeal Carcinoma. PLoS One 2016;11:e0157969. [Crossref] [PubMed]
- Li YF, Ding JW, Liao LM, et al. Expression of programmed death ligand-1 predicts poor outcome in nasopharyngeal carcinoma. Mol Clin Oncol 2017;7:378-82. [Crossref] [PubMed]
- Fang W, Zhang J, Hong S, et al. EBV-driven LMP1 and IFN-γ up-regulate PD-L1 in nasopharyngeal carcinoma: Implications for oncotargeted therapy. Oncotarget 2014;5:12189-202. [Crossref] [PubMed]
- Qu Y, Wang D, Yang L, et al. Expression and clinical significance of programmed death ligand 1 in nasopharyngeal carcinoma. Mol Clin Oncol 2018;9:75-81. [Crossref] [PubMed]
- Zheng L, Cao C, Cheng G, et al. Cytomembranic PD-L1 expression in locoregionally advanced nasopharyngeal carcinoma. Onco Targets Ther 2017;10:5483-7. [Crossref] [PubMed]
- Zhou Y, Miao J, Wu H, et al. PD-1 and PD-L1 expression in 132 recurrent nasopharyngeal carcinoma: the correlation with anemia and outcomes. Oncotarget 2017;8:51210-23. [Crossref] [PubMed]
- Zhou Y, Shi D, Miao J, et al. PD-L1 predicts poor prognosis for nasopharyngeal carcinoma irrespective of PD-1 and EBV-DNA load. Sci Rep 2017;7:43627. [Crossref] [PubMed]
- Liu YJ, Tsang NM, Hsueh C, et al. Low PD-L1 Expression Strongly Correlates with Local Recurrence in Epstein-Barr Virus-Positive Nasopharyngeal Carcinoma after Radiation-Based Therapy. Cancers (Basel) 2018;10:374. [Crossref] [PubMed]
- Tang Y, He Y, Shi L, et al. Co-expression of AFAP1-AS1 and PD-1 predicts poor prognosis in nasopharyngeal carcinoma. Oncotarget 2017;8:39001-11. [Crossref] [PubMed]
- Liou AK, Soon G, Tan L, et al. Elevated IL18 levels in Nasopharyngeal carcinoma induced PD-1 expression on NK cells in TILS leading to poor prognosis. Oral Oncol 2020;104:104616. [Crossref] [PubMed]
- Jiang F, Yu W, Zeng F, et al. PD-1 high expression predicts lower local disease control in stage IV M0 nasopharyngeal carcinoma. BMC Cancer 2019;19:503. [Crossref] [PubMed]
- Hsu MC, Hsiao JR, Chang KC, et al. Increase of programmed death-1-expressing intratumoral CD8 T cells predicts a poor prognosis for nasopharyngeal carcinoma. Mod Pathol 2010;23:1393-403. [Crossref] [PubMed]
- Chang CS, Chang JH, Hsu NC, et al. Expression of CD80 and CD86 costimulatory molecules are potential markers for better survival in nasopharyngeal carcinoma. BMC Cancer 2007;7:88. [Crossref] [PubMed]
- Zhang G, Xu Y, Zhou H. The Infiltration of ICOS+ Cells in Nasopharyngeal Carcinoma is Beneficial for Improved Prognosis. Pathol Oncol Res 2020;26:365-70. [Crossref] [PubMed]
- Ooft ML, van Ipenburg JA, Sanders ME, et al. Prognostic role of tumour-associated macrophages and regulatory T cells in EBV-positive and EBV-negative nasopharyngeal carcinoma. J Clin Pathol 2018;71:267-74. [Crossref] [PubMed]
- Xu T, Huang Z, Su B, et al. Prognostic significance of circulating CD19+ B lymphocytes in EBV-associated nasopharyngeal carcinoma. Med Oncol 2014;31:198. [Crossref] [PubMed]
- Yang Y, Zhang P, Zhang H, et al. Immunocyte infiltration characteristics of gene expression profile in nasopharyngeal carcinoma and clinical significance. Xi Bao Yu Fen Zi Mian Yi Xue Za Zhi 2020;36:1069-75. [PubMed]
- Chen L, Li M, Xu T, et al. Prognostic value of neutrophils for patients with nasopharyngeal carcinoma. Laryngoscope Investig Otolaryngol 2021;6:219-25. [Crossref] [PubMed]
- Chen X, Li X, Zhao F, et al. Distribution and prognostic significance of tumor-infiltrating mast cells in nasopharyngeal carcinoma. Zhonghua Er Bi Yan Hou Tou Jing Wai Ke Za Zhi 2015;50:306-11. [PubMed]
- Goldman SA, Baker E, Weyant RJ, et al. Peritumoral CD1a-positive dendritic cells are associated with improved survival in patients with tongue carcinoma. Arch Otolaryngol Head Neck Surg 1998;124:641-6. [Crossref] [PubMed]
- Gallo O, Bianchi S, Giannini A, et al. Correlations between histopathological and biological findings in nasopharyngeal carcinoma and its prognostic significance. Laryngoscope 1991;101:487-93. [Crossref] [PubMed]
- Giannini A, Bianchi S, Messerini L, et al. Prognostic significance of accessory cells and lymphocytes in nasopharyngeal carcinoma. Pathol Res Pract 1991;187:496-502. [Crossref] [PubMed]
- Takenaka Y, Kitamura T, Oya R, et al. Prognostic role of neutrophil-lymphocyte ratio in nasopharyngeal carcinoma: A meta-analysis. PLoS One 2017;12:e0181478. [Crossref] [PubMed]
- Chen C, Sun P, Dai QS, et al. The Glasgow Prognostic Score predicts poor survival in cisplatin-based treated patients with metastatic nasopharyngeal carcinoma. PLoS One 2014;9:e112581. [Crossref] [PubMed]
- Jin Y, Ye X, He C, et al. Pretreatment neutrophil-to-lymphocyte ratio as predictor of survival for patients with metastatic nasopharyngeal carcinoma. Head Neck 2015;37:69-75. [Crossref] [PubMed]
- Zergoun AA, Zebboudj A, Sellam SL, et al. IL-6/NOS2 inflammatory signals regulate MMP-9 and MMP-2 activity and disease outcome in nasopharyngeal carcinoma patients. Tumour Biol 2016;37:3505-14. [Crossref] [PubMed]
- Zhang Y, Sun H, Wu H, et al. Interleukin 35 is an independent prognostic factor and a therapeutic target for nasopharyngeal carcinoma. Contemp Oncol (Pozn) 2015;19:120-4. [Crossref] [PubMed]
- Meseure D, Drak Alsibai K, Nicolas A. Pivotal role of pervasive neoplastic and stromal cells reprogramming in circulating tumor cells dissemination and metastatic colonization. Cancer Microenviron 2014;7:95-115. [Crossref] [PubMed]
- Drak Alsibai K, Meseure D. Tumor microenvironment and noncoding RNAs as co-drivers of epithelial-mesenchymal transition and cancer metastasis. Dev Dyn 2018;247:405-31. [Crossref] [PubMed]
- Huang SCM, Tsao SW, Tsang CM. Interplay of Viral Infection, Host Cell Factors and Tumor Microenvironment in the Pathogenesis of Nasopharyngeal Carcinoma. Cancers (Basel) 2018;10:106. [Crossref] [PubMed]
- Huang YT, Sheen TS, Chen CL, et al. Profile of cytokine expression in nasopharyngeal carcinomas: a distinct expression of interleukin 1 in tumor and CD4+ T cells. Cancer Res 1999;59:1599-605. [PubMed]
- Sarwari NM, Khoury JD, Hernandez CM. Chronic Epstein Barr virus infection leading to classical Hodgkin lymphoma. BMC Hematol 2016;16:19. [Crossref] [PubMed]
- Guo W, Giancotti FG. Integrin signalling during tumour progression. Nat Rev Mol Cell Biol 2004;5:816-26. [Crossref] [PubMed]
- Shimizu Y, Shaw S. Lymphocyte interactions with extracellular matrix. FASEB J 1991;5:2292-9. [Crossref] [PubMed]
- Gong L, Kwong DL, Dai W, et al. The Stromal and Immune Landscape of Nasopharyngeal Carcinoma and Its Implications for Precision Medicine Targeting the Tumor Microenvironment. Front Oncol 2021;11:744889. [Crossref] [PubMed]
- Ghajar CM, Peinado H, Mori H, et al. The perivascular niche regulates breast tumour dormancy. Nat Cell Biol 2013;15:807-17. [Crossref] [PubMed]
- Navarro R, Compte M, Álvarez-Vallina L, et al. Immune Regulation by Pericytes: Modulating Innate and Adaptive Immunity. Front Immunol 2016;7:480. [Crossref] [PubMed]
- Beckermann BM, Kallifatidis G, Groth A, et al. VEGF expression by mesenchymal stem cells contributes to angiogenesis in pancreatic carcinoma. Br J Cancer 2008;99:622-31. [Crossref] [PubMed]
- Ge S, Mao Y, Yi Y, et al. Comparative proteomic analysis of secreted proteins from nasopharyngeal carcinoma-associated stromal fibroblasts and normal fibroblasts. Exp Ther Med 2012;3:857-60. [Crossref] [PubMed]
- Nurmik M, Ullmann P, Rodriguez F, et al. In search of definitions: Cancer-associated fibroblasts and their markers. Int J Cancer 2020;146:895-905. [Crossref] [PubMed]
- Khan GJ, Sun L, Khan S, et al. Versatility of Cancer Associated Fibroblasts: Commendable Targets for Anti-tumor Therapy. Curr Drug Targets 2018;19:1573-88. [Crossref] [PubMed]
- Turley SJ, Cremasco V, Astarita JL. Immunological hallmarks of stromal cells in the tumour microenvironment. Nat Rev Immunol 2015;15:669-82. [Crossref] [PubMed]
- Sautès-Fridman C, Lawand M, Giraldo NA, et al. Tertiary Lymphoid Structures in Cancers: Prognostic Value, Regulation, and Manipulation for Therapeutic Intervention. Front Immunol 2016;7:407. [Crossref] [PubMed]
- Gourzones C, Barjon C, Busson P. Host-tumor interactions in nasopharyngeal carcinomas. Semin Cancer Biol 2012;22:127-36. [Crossref] [PubMed]
- Lanier LL. NKG2D Receptor and Its Ligands in Host Defense. Cancer Immunol Res 2015;3:575-82. [Crossref] [PubMed]
- Jin YY, Yang WZ, Zou S, et al. Chemoradiotherapy combined with NK cell transfer in a patient with recurrent and metastatic nasopharyngeal carcinoma inducing long-term tumor control: A case report. Medicine (Baltimore) 2020;99:e22785. [Crossref] [PubMed]
- Hadrup S, Donia M, Thor Straten P. Effector CD4 and CD8 T cells and their role in the tumor microenvironment. Cancer Microenviron 2013;6:123-33. [Crossref] [PubMed]
- Pandiyan P, Conti HR, Zheng L, et al. CD4(+)CD25(+)Foxp3(+) regulatory T cells promote Th17 cells in vitro and enhance host resistance in mouse Candida albicans Th17 cell infection model. Immunity 2011;34:422-34. [Crossref] [PubMed]
- Rossi JF, Céballos P, Lu ZY. Immune precision medicine for cancer: a novel insight based on the efficiency of immune effector cells. Cancer Commun (Lond) 2019;39:34. [Crossref] [PubMed]
- Nicholls J, Niedobitek G. Histopathological Diagnosis of Nasopharyngeal Carcinoma: Looking beyond the Blue Book. In: Busson P (ed.). Nasopharyngeal Carcinoma. New York, NY: Springer New York, 2013:10-22.
- Zanussi S, Vaccher E, Caffau C, et al. Interferon-gamma secretion and perforin expression are impaired in CD8+ T lymphocytes from patients with undifferentiated carcinoma of nasopharyngeal type. Cancer Immunol Immunother 2003;52:28-32. [Crossref] [PubMed]
- Li J, Zeng XH, Mo HY, et al. Functional inactivation of EBV-specific T-lymphocytes in nasopharyngeal carcinoma: implications for tumor immunotherapy. PLoS One 2007;2:e1122. [Crossref] [PubMed]
- Baumforth KR, Birgersdotter A, Reynolds GM, et al. Expression of the Epstein-Barr virus-encoded Epstein-Barr virus nuclear antigen 1 in Hodgkin’s lymphoma cells mediates Up-regulation of CCL20 and the migration of regulatory T cells. Am J Pathol 2008;173:195-204. [Crossref] [PubMed]
- Chang KP, Chang YT, Wu CC, et al. Multiplexed immunobead-based profiling of cytokine markers for detection of nasopharyngeal carcinoma and prognosis of patient survival. Head Neck 2011;33:886-97. [Crossref] [PubMed]
- Li J, Huang ZF, Xiong G, et al. Distribution, characterization, and induction of CD8+ regulatory T cells and IL-17-producing CD8+ T cells in nasopharyngeal carcinoma. J Transl Med 2011;9:189. [Crossref] [PubMed]
- Beck A, Päzolt D, Grabenbauer GG, et al. Expression of cytokine and chemokine genes in Epstein-Barr virus-associated nasopharyngeal carcinoma: comparison with Hodgkin’s disease. J Pathol 2001;194:145-51. [Crossref] [PubMed]
- Tan EL, Selvaratnam G, Kananathan R, et al. Quantification of Epstein-Barr virus DNA load, interleukin-6, interleukin-10, transforming growth factor-beta1 and stem cell factor in plasma of patients with nasopharyngeal carcinoma. BMC Cancer 2006;6:227. [Crossref] [PubMed]
- Wang YQ, Chen YP, Zhang Y, et al. Prognostic significance of tumor-infiltrating lymphocytes in nondisseminated nasopharyngeal carcinoma: A large-scale cohort study. Int J Cancer 2018;142:2558-66. [Crossref] [PubMed]
- Neyt K, Perros F. Tertiary lymphoid organs in infection and autoimmunity. Trends Immunol 2012;33:297-305. [Crossref] [PubMed]
- Dieu-Nosjean MC, Goc J, Giraldo NA, et al. Tertiary lymphoid structures in cancer and beyond. Trends Immunol 2014;35:571-80. [Crossref] [PubMed]
- Legler DF, Loetscher M, Roos RS, et al. B cell-attracting chemokine 1, a human CXC chemokine expressed in lymphoid tissues, selectively attracts B lymphocytes via BLR1/CXCR5. J Exp Med 1998;187:655-60. [Crossref] [PubMed]
- Armas-González E, Domínguez-Luis MJ, Díaz-Martín A, et al. Role of CXCL13 and CCL20 in the recruitment of B cells to inflammatory foci in chronic arthritis. Arthritis Res Ther 2018;20:114. [Crossref] [PubMed]
- Bindea G, Mlecnik B, Tosolini M, et al. Spatiotemporal dynamics of intratumoral immune cells reveal the immune landscape in human cancer. Immunity 2013;39:782-95. [Crossref] [PubMed]
- Song IH, Heo SH, Bang WS, et al. Predictive Value of Tertiary Lymphoid Structures Assessed by High Endothelial Venule Counts in the Neoadjuvant Setting of Triple-Negative Breast Cancer. Cancer Res Treat 2017;49:399-407. [Crossref] [PubMed]
- Li JP, Wu CY, Chen MY, et al. PD-1+CXCR5-CD4+ Th-CXCL13 cell subset drives B cells into tertiary lymphoid structures of nasopharyngeal carcinoma. J Immunother Cancer 2021;9:e002101. [Crossref] [PubMed]
- Shurin MR, Shurin GV, Lokshin A, et al. Intratumoral cytokines/chemokines/growth factors and tumor infiltrating dendritic cells: friends or enemies? Cancer Metastasis Rev 2006;25:333-56. [Crossref] [PubMed]
- Colonna M, Trinchieri G, Liu YJ. Plasmacytoid dendritic cells in immunity. Nat Immunol 2004;5:1219-26. [Crossref] [PubMed]
- Nilsson JS, Abolhalaj M, Lundberg K, et al. Dendritic cell subpopulations in nasopharyngeal cancer. Oncol Lett 2019;17:2557-61. [PubMed]
- Teichmann M, Meyer B, Beck A, et al. Expression of the interferon-inducible chemokine IP-10 (CXCL10), a chemokine with proposed anti-neoplastic functions, in Hodgkin lymphoma and nasopharyngeal carcinoma. J Pathol 2005;206:68-75. [Crossref] [PubMed]
- Hu H, Tang KF, Chua YN, et al. Expression of interleukin-18 by nasopharyngeal carcinoma cells: a factor that possibly initiates the massive leukocyte infiltration. Hum Pathol 2004;35:722-8. [Crossref] [PubMed]
- Qian BZ, Pollard JW. Macrophage diversity enhances tumor progression and metastasis. Cell 2010;141:39-51. [Crossref] [PubMed]
- Chimal-Ramírez GK, Espinoza-Sánchez NA, Fuentes-Pananá EM. Protumor activities of the immune response: insights in the mechanisms of immunological shift, oncotraining, and oncopromotion. J Oncol 2013;2013:835956. [Crossref] [PubMed]
- Yoshimura T. Discovery of IL-8/CXCL8 (The Story from Frederick). Front Immunol 2015;6:278. [Crossref] [PubMed]
- Mizuno R, Kawada K, Itatani Y, et al. The Role of Tumor-Associated Neutrophils in Colorectal Cancer. Int J Mol Sci 2019;20:529. [Crossref] [PubMed]
- van der Windt DJ, Sud V, Zhang H, et al. Neutrophil extracellular traps promote inflammation and development of hepatocellular carcinoma in nonalcoholic steatohepatitis. Hepatology 2018;68:1347-60. [Crossref] [PubMed]
- Lee CH, Yeh TH, Lai HC, et al. Epstein-Barr virus Zta-induced immunomodulators from nasopharyngeal carcinoma cells upregulate interleukin-10 production from monocytes. J Virol 2011;85:7333-42. [Crossref] [PubMed]
- Lippitz BE. Cytokine patterns in patients with cancer: a systematic review. Lancet Oncol 2013;14:e218-28. [Crossref] [PubMed]
- Hsu M, Wu SY, Chang SS, et al. Epstein-Barr virus lytic transactivator Zta enhances chemotactic activity through induction of interleukin-8 in nasopharyngeal carcinoma cells. J Virol 2008;82:3679-88. [Crossref] [PubMed]
- Pan XB, Huang ST, Zhu XD. Neutrophil-to-lymphocyte ratio predicts the prognosis of stage II nasopharyngeal carcinoma. Cancer Manag Res 2019;11:8269-75. [Crossref] [PubMed]
- Hsu C, Lee SH, Ejadi S, et al. Safety and Antitumor Activity of Pembrolizumab in Patients With Programmed Death-Ligand 1-Positive Nasopharyngeal Carcinoma: Results of the KEYNOTE-028 Study. J Clin Oncol 2017;35:4050-6. [Crossref] [PubMed]
- Ma BBY, Lim WT, Goh BC, et al. Antitumor Activity of Nivolumab in Recurrent and Metastatic Nasopharyngeal Carcinoma: An International, Multicenter Study of the Mayo Clinic Phase 2 Consortium (NCI-9742). J Clin Oncol 2018;36:1412-8. [Crossref] [PubMed]
- Fang W, Yang Y, Ma Y, et al. Camrelizumab (SHR-1210) alone or in combination with gemcitabine plus cisplatin for nasopharyngeal carcinoma: results from two single-arm, phase 1 trials. Lancet Oncol 2018;19:1338-50. [Crossref] [PubMed]
- Iglesia MD, Parker JS, Hoadley KA, et al. Genomic Analysis of Immune Cell Infiltrates Across 11 Tumor Types. J Natl Cancer Inst 2016; [Crossref] [PubMed]
- Roh W, Chen PL, Reuben A, et al. Integrated molecular analysis of tumor biopsies on sequential CTLA-4 and PD-1 blockade reveals markers of response and resistance. Sci Transl Med 2017;9:eaah3560. [Crossref] [PubMed]
- Thorsson V, Gibbs DL, Brown SD, et al. The Immune Landscape of Cancer. Immunity 2018;48:812-830.e14. [Crossref] [PubMed]
- Chen YP, Wang YQ, Lv JW, et al. Identification and validation of novel microenvironment-based immune molecular subgroups of head and neck squamous cell carcinoma: implications for immunotherapy. Ann Oncol 2019;30:68-75. [Crossref] [PubMed]
- Codina A, Renauer PA, Wang G, et al. Convergent Identification and Interrogation of Tumor-Intrinsic Factors that Modulate Cancer Immunity In Vivo. Cell Syst 2019;8:136-151.e7. [Crossref] [PubMed]
- Zhu S, Qing T, Zheng Y, et al. Advances in single-cell RNA sequencing and its applications in cancer research. Oncotarget 2017;8:53763-79. [Crossref] [PubMed]
- Ren X, Kang B, Zhang Z. Understanding tumor ecosystems by single-cell sequencing: promises and limitations. Genome Biol 2018;19:211. [Crossref] [PubMed]
- Wang YA, Li XL, Mo YZ, et al. Effects of tumor metabolic microenvironment on regulatory T cells. Mol Cancer 2018;17:168. [Crossref] [PubMed]
- Wu T, Wu X, Wang HY, et al. Immune contexture defined by single cell technology for prognosis prediction and immunotherapy guidance in cancer. Cancer Commun (Lond) 2019;39:21. [Crossref] [PubMed]
- Mints M, Tirosh I. Nasopharyngeal carcinoma joins the single-cell party. Cancer Commun (Lond) 2020;40:453-5. [Crossref] [PubMed]
- Zhao M, Luo R, Liu Y, et al. miR-3188 regulates nasopharyngeal carcinoma proliferation and chemosensitivity through a FOXO1-modulated positive feedback loop with mTOR-p-PI3K/AKT-c-JUN. Nat Commun 2016;7:11309. [Crossref] [PubMed]
- Zhang Y, Hu CF, Chen J, et al. LATS2 is de-methylated and overexpressed in nasopharyngeal carcinoma and predicts poor prognosis. BMC Cancer 2010;10:538. [Crossref] [PubMed]
- Huang J, Huang W, Liu M, et al. Enhanced expression of SETDB1 possesses prognostic value and promotes cell proliferation, migration and invasion in nasopharyngeal carcinoma. Oncol Rep 2018;40:1017-25. [Crossref] [PubMed]
- Teng Y, Hu L, Yu B, et al. Cytoplasmic p27 is a novel prognostic biomarker and oncogenic protein for nasopharyngeal carcinoma. Artif Cells Nanomed Biotechnol 2020;48:336-44. [Crossref] [PubMed]
- Chen C, Lu Z, Yang J, et al. MiR-17-5p promotes cancer cell proliferation and tumorigenesis in nasopharyngeal carcinoma by targeting p21. Cancer Med 2016;5:3489-99. [Crossref] [PubMed]
- Li A, Shi D, Xu B, et al. S100A6 promotes cell proliferation in human nasopharyngeal carcinoma via the p38/MAPK signaling pathway. Mol Carcinog 2017;56:972-84. [Crossref] [PubMed]
- Mai J, Zhong ZY, Guo GF, et al. Polo-Like Kinase 1 phosphorylates and stabilizes KLF4 to promote tumorigenesis in nasopharyngeal carcinoma. Theranostics 2019;9:3541-54. [Crossref] [PubMed]
- Otto T, Sicinski P. Cell cycle proteins as promising targets in cancer therapy. Nat Rev Cancer 2017;17:93-115. [Crossref] [PubMed]
- Zhao J, Guo C, Xiong F, et al. Single cell RNA-seq reveals the landscape of tumor and infiltrating immune cells in nasopharyngeal carcinoma. Cancer Lett 2020;477:131-43. [Crossref] [PubMed]
- Chen YP, Yin JH, Li WF, et al. Single-cell transcriptomics reveals regulators underlying immune cell diversity and immune subtypes associated with prognosis in nasopharyngeal carcinoma. Cell Res 2020;30:1024-42. [Crossref] [PubMed]
- Chen YP, Chan ATC, Le QT, et al. Nasopharyngeal carcinoma. Lancet 2019;394:64-80. [Crossref] [PubMed]
- Dehghani M, Sharifpour S, Amirghofran Z, et al. Prognostic significance of T cell subsets in peripheral blood of B cell non-Hodgkin’s lymphoma patients. Med Oncol 2012;29:2364-71. [Crossref] [PubMed]
- Hu FJ, Ge MH, Li P, et al. Unfavorable clinical implications of circulating CD44+ lymphocytes in patients with nasopharyngeal carcinoma undergoing radiochemotherapy. Clin Chim Acta 2012;413:213-8. [Crossref] [PubMed]
- Schmidt MA, Förtsch C, Schmidt M, et al. Circulating regulatory T cells of cancer patients receiving radiochemotherapy may be useful to individualize cancer treatment. Radiother Oncol 2012;104:131-8. [Crossref] [PubMed]
- Shah W, Yan X, Jing L, et al. A reversed CD4/CD8 ratio of tumor-infiltrating lymphocytes and a high percentage of CD4(+)FOXP3(+) regulatory T cells are significantly associated with clinical outcome in squamous cell carcinoma of the cervix. Cell Mol Immunol 2011;8:59-66. [Crossref] [PubMed]
- Chen YP, Lv JW, Mao YP, et al. Unraveling tumour microenvironment heterogeneity in nasopharyngeal carcinoma identifies biologically distinct immune subtypes predicting prognosis and immunotherapy responses. Mol Cancer 2021;20:14. [Crossref] [PubMed]
- Gong L, Kwong DL, Dai W, et al. Comprehensive single-cell sequencing reveals the stromal dynamics and tumor-specific characteristics in the microenvironment of nasopharyngeal carcinoma. Nat Commun 2021;12:1540. [Crossref] [PubMed]
- Jin S, Li R, Chen MY, et al. Single-cell transcriptomic analysis defines the interplay between tumor cells, viral infection, and the microenvironment in nasopharyngeal carcinoma. Cell Res 2020;30:950-65. [Crossref] [PubMed]
- Avila Cobos F, Vandesompele J, Mestdagh P, et al. Computational deconvolution of transcriptomics data from mixed cell populations. Bioinformatics 2018;34:1969-79. [Crossref] [PubMed]
- Ding RB, Chen P, Rajendran BK, et al. Molecular landscape and subtype-specific therapeutic response of nasopharyngeal carcinoma revealed by integrative pharmacogenomics. Nat Commun 2021;12:3046. [Crossref] [PubMed]
- Asp M, Bergenstråhle J, Lundeberg J. Spatially Resolved Transcriptomes-Next Generation Tools for Tissue Exploration. Bioessays 2020;42:e1900221. [Crossref] [PubMed]
- Ji AL, Rubin AJ, Thrane K, et al. Multimodal Analysis of Composition and Spatial Architecture in Human Squamous Cell Carcinoma. Cell 2020;182:497-514.e22. [Crossref] [PubMed]
Cite this article as: Liu SX, Zhao GX, Lin RB, Zeng MS, Zhong Q. Classifying the tumor microenvironment to stratify nasopharyngeal carcinoma patients. Ann Nasopharynx Cancer 2022;6:8.